Abstract
Context: Calorie restriction reduces circulating triiodothyronine (T3) – the most active thyroid hormone – inducing hypothyroidism, constipation, and reduced appetite that inhibit eating, acting to sustain and sometimes precipitate eating disorders. Thyroid-hormone treatment can be effective but is rarely employed.
Case description: A 12-year-old girl experienced chronic nausea and constipation after antibiotics for strep throat, resulting in restricted eating. Energy decreased for 2 months as anxiety and depression increased, culminating in withdrawal and school refusal. Blood tests were normal except for below-range free T3. She began a trial of incremental, 1-μg dose increases of T3 every 3 days using sustained-release capsules added to her established triiodothyronine–levothyroxine (T3–T4) protocol for hypothyroidism. Her energy and mood began improving after 10 days and depression resolved after 7 weeks. An 18-day stay in a hospital eating-disorders unit helped her regain some weight and strength. To address nausea and anxiety, the extended medical team then tried two antidepressants and cyproheptadine – each caused increased nausea and reduced eating – and a 5-day prednisone burst for possible neuroinflammation; all were ineffective and discontinued. After 14 weeks without improvement, we resumed adjusting thyroid-hormone dose. Anxiety, energy, food intake, and weight improved for 5 months. She began attending a new school, developed new friendships, and started menses. The major illness lasted 21 months. T3 intake increased from 5 to 27 μg, T4 from 50 to 110 μg, weight from 36.4 to 52.7 kg, and height from 1.63 to 1.71 m.
Conclusions: Thyroid hormones should be studied further as fundamentally effective treatment for eating disorders.
Keywords: Eating disorder; Low-triiodothyronine syndrome; Hypothyroidism
INTRODUCTION
Thyroid Hormones Are Essential For Normal metabolism, growth and maturation, brain development and maintenance, immune function, and various signal transduction pathways.1-4 The healthy thyroid gland produces small amounts of active triiodothyronine (T3) and larger amounts of levothyroxine (T4), its biologically inactive prohormone. Hypothyroidism Results From Insufficient T3 reaching and stimulating nuclear receptors within cells, causing low metabolism, fatigue, and body-wide dysfunction. Mental, psychological, and neurological disturbance may include impaired concentration, memory and executive functioning, insomnia, anxiety, depression, social awkwardness, withdrawal, perseveration, paranoia, agitated delirium, full psychosis, and altered perceptions (taste, hearing, vision).5-9 Other common signs and symptoms include dry skin, weak fingernails, joint and muscle pain, muscle cramps, hair loss, cold intolerance, menstrual irregularities, arrhythmias, and elevated cholesterol and blood pressure.5-14
Circulating T3 levels decrease in eating disorders (EDs) and in most severe and chronic illnesses in the eponymous medical condition low-T3 syndrome (LT3S), also known as non-thyroidal illness syndrome (NTIS).15,16 Thyroid-hormone signaling changes differentially among tissues with an overall reduction of metabolic rate, indicating hypothyroidism.15,17 LT3S occurs broadly in severe and chronic illnesses including trauma, sepsis, heart failure, COVID-19, and during calorie restriction aside from EDs.10,18-21 Lower serum T3 level is prognostic of increased disease severity and poor outcomes, including death.15,20-28 LT3S has major roles in sustaining eating disorders by causing chronic constipation that inhibits eating and weight gain.29,30 T3 also acts directly on the hypothalamus to stimulate feeding (independent of energy expenditure). Reduced circulating T3 in LT3S is likely a factor in appetite suppression.31-34 LT3S may also cause or exacerbate any of the mental and physical disturbances of hypothyroidism described above, and it contributes to the long-term health consequences of EDs such as delayed puberty and growth, and osteoporosis.35-40 Though thyroid-hormone dysfunction is central to EDs, thyroid-hormone treatment is rarely considered by doctors or presented as an option to patients. However, ED patients urgently need new, effective therapies: only about 50% respond to first-line treatments, and mortality is high.41 This case study demonstrates the ability of thyroid hormone therapy to help resolve eating disorders by fundamentally addressing motility, hunger, eating patterns, and hormone-associated psychological disturbance.
Reasons To Focus On Low T3 Syndrome (LT3S)
In contrast to primary hypothyroidism, where hormone production by the thyroid gland is reduced, LT3S begins with enhanced peripheral inactivation of T4 and T3.42 The balance between activation and deactivation is coordinated by a set of deiodinase enzymes within cells: T4 is activated by conversion to T3 or inactivated by conversion to reverse T3 (rT3), which is often elevated in LT3S. T3 is inactivated by conversion to 3,3′-T2 (3,3′-diiodothyronine).15,17,43-47 LT3S also involves changes in thyroid hormone transporters, membrane transport, and receptors.46,48 In severe or prolonged LT3S, thyroid stimulating hormone (TSH) and T4 can also decrease, reducing T3 further.16,46,47,49
By the 1970s, low serum T3 was recognized as a factor in eating disorders, and oral T3 had shown potential for treatment.38 However, follow up studies have been lacking, in the ED field as well as in other low-T3 health conditions. As described by prominent endocrinologists, progress in thyroid treatment has been hampered by the following seismic changes in the field that began in the 1970s50-54:
- Treatment with T3 and T3/T4 fell out of favor while T4 monotherapy become dominant:
For 50 years now, providers have been steered away from using anything other than LT4 in the treatment of hypothyroidism. This has resulted in two generations of physicians with limited clinical experience prescribing LT3 or DTE,1 and with the taught perception that these drugs were unsafe. This constitutes a major challenge for those willing to follow the guidelines and try combination therapy for specific situations.52 - Diagnosis and monitoring of thyroid dysfunction shifted to rely heavily on biochemical testing - mainly of TSH - rather that resolving the signs and symptoms of individual patients to restore their health and quality of life:
Over-reliance on TSH As A gold standard has long impeded the advancement of the field, since the first doubts were raised, and disagreements emerged on the setting of the reference intervals. While sole reliance on TSH must therefore be scaled back, good clinical practice taking into account the full history and symptoms displayed by a patient has to be reinstituted as a primary tool.44 It appears that we are witnessing a consequential historic shift in the treatment of thyroid disease, driven by over-reliance on a single laboratory parameter, TSH. The focus on biochemistry rather than patient symptom relief should be re-assessed. A joint consideration together with a more personalized approach may be required to address the recent surge in patient complaint rates.50
These issues have been noticed broadly and prompted institutional change. In 2021, the American, British, And European Thyroid Associations published a consensus document that called for “better designed trials” of T3/T4 combination therapy “focusing on clinically relevant outcomes, including patient-centered outcomes,” where T3 will be multi-dosed or given in sustainedrelease (SR) form if available.54 They noted how progress in T3/T4 therapy has been impeded by many poorly designed, cookie-cutter T3/T4 studies that failed to show consistent patient benefit.
Low T3 is also increasingly recognized as a central factor in the progression and severity of acute and chronic illnesses,20,46,47,55,56 and new clinical trials are investigating T3 treatment in conditions such as heart failure56-58 and COVID-19.19,59 (Notably, early results from the T3 COVID-19 trial show reduction of sedimentation rate, a marker of inflammation.59)
T4 and T3 Therapy for Pre-Existing Hypothyroidism
Individuals diagnosed with hypothyroidism are nearly always treated with T4 monotherapy; however, a significant fraction - usually stated as 10-15% in the literature - respond poorly and are left with fatigue and other residual symptoms. The causes of poor response are under study and include the T4 hormone-replacement approach itself, which is unphysiological, as well as genetic factors, endocrine disrupting compounds, and hormone resistance acquired from stress or illness.46,47,51,60-62
Oral T4 monotherapy differs from endogenous production in chemical composition, rate and route of absorption, and resulting serum levels of T4 and T3. The healthy thyroid gland meters T4 and T3 directly and steadily into the blood stream with a circadian rise of T3 in the early morning63 and with T3 production ranging from around 6% to 42 % that of T4 among patients, according to an analysis of a 1990 study by Pilo et al.64,65 Healthy individuals maintain remarkably steady circulating T3 level as a biological priority via an intricate balancing act that involves central and local control, and feedback and feedforward mechanisms.43,44,51,66,67 TSH varies with time and circumstance to support this steady T3 level, through negative feedback in the hypothalamic-pituitary-thyroid (HPT) axis and de novo synthesis in the thyroid gland, and through feedforward, whereby bloodborne T4 is converted more efficiently to T3 as it circulates through the thyroid gland. T4-to-T3 conversion is catalyzed by D1 and D2, two of the three deiodinase enzymes that control the metabolism of thyroid hormones within cells. D1 is expressed primarily in the liver and kidneys, while D2 is expressed in the thyroid gland, skeletal muscle, brain, and other tissues.68,69 TSH increases D2 activity, especially in the thyroid gland, causing it to produce more T3, which positively regulates D1 to further increase T3 production.43,44,51,66,67 Evidence suggests that TSH may act directly on thyroidal D2 and D1 to produce the bulk of this feedforward enhancement of T3 production.70,71 The Third Deiodinase Enzyme, D3, deactivates excess T3 and T4 to maintain steady T3 in coordination with D1 and D2.44,68
Many downstream markers of hypothyroidism are not normalized by T4 monotherapy, including abnormal cholesterol and lipid levels, and liver and kidney function.54,75,76 Hypothyroid patients are disposed to impaired gastrointestinal (GI) motility and constipation that underly eating disorders,29,30 And T4 therapy is a strong predictor of developing small-intestinal bacterial overgrowth (SIBO) - stronger than hypothyroidism or impaired intestinal clearance alone - which occurs frequently in eating disorders.77 Rapid absorption of oral T4 into the liver and other tissues may have substantial negative health consequences that have been largely overlooked. Plasma T4 rises and peaks a few hours after ingestion of an oral dose.78 Liver T4 concentration can be expected to rise more quickly and to a higher level than in the systemic circulation by simple compartmental analysis: the full T4 dose enters through the portal vein and liver, which together have much smaller volume than the systemic circulation (approximately 10 times lower). The liver must necessarily have a higher peak concentration that is then diluted into the much larger systemic volume as the two compartments equilibrate.
The liver and other tissues can express deiodinase type 3 (D3) to deactivate excess T3 and T4 and prevent hypermetabolism.44,68 D3 is positively regulated by T3 and T4,43,45,49,68,69,79,80 therefore an oral T4 dose entering the liver at high concentration should increase liver D3 activity and, with it, the inactivation of local T4 and T3.2 Lower T3 will in turn reduce D1 activity, causing a further decrease in T3 and disposing the patient to hypothyroidism.
This self-reinforcing, T3-suppressive cycle may help explain the low T3 levels observed in T4 monotherapy and patient complaints of residual symptoms. These effects may be enhanced in illness and calorie restriction, where liver expression of D1 decreases while that of D3 increases.82 (There are also case reports of outright liver injury due to T4 therapy.83-85)
These mechanisms should also be involved in the body’s response to rapid-release T3/T4 and T3 therapy and may explain related clinical observations.
First, many patients feel best at a high circulating T3 level, sometimes above the reference range.
They may need sufficient T3 to counter liver deactivation and raise metabolism. Second, some patients on a T4-only protocol feel tremendous benefit from adding small, microgram doses of T3. The minuscule T3 dose may increase liver D1 activity and T4-to-T3 conversion without appreciably increasing D3 activity and inactivation of T3 and T4.
First-pass liver metabolism of rapid-release T4 or T3 may also dispose patients to hypocortisolism, which can be debilitating and dangerous. Thyroidhormone products carry a contraindication warning in uncorrected adrenal insufficiency because they increase metabolic clearance of glucocorticoids.86 However, hypocortisolism is also experienced by a substantial number of hypothyroid patients on thyroid-hormone therapy who had no prior history or indication of adrenal dysfunction. Thyroid hormones, especially T3, have a dual effect of increasing the release of cortisol by the adrenal glands and its clearance by liver, and both hypothyroidism and hyperthyroidism cause abnormal cortisol levels.87-92 Sufficient cortisol is also needed for T3 to bind and activate nuclear receptors,3 but excess cortisol reduces T3 levels (which is responsible in part for decreased immune function due to stress).47,94 Dysregulation of cortisol is a factor in eating disorders (as is dysregulation of steroids and other endogenous hormones more broadly).95-97
Thyroid-hormone therapy must support balance between cortisol production and clearance to optimize health and wellbeing. For many patients, this balance is difficult to attain with rapid-release T4 and T3, likely due to or exacerbated by first-pass liver metabolism.
Multi-dosing and sustained-release formulations help, as we will discuss.
A prescription of hydrocortisone (cortisol) or synthetic corticosteroid may be warranted.8,98-100 Pregnenolone is an over-the-counter precursor of cortisol that can sometimes be used effectively in place of hydrocortisone. Patients have also found that taking T3 in the morning around 4-5 am can increase cortisol release to normal levels, allowing them to discontinue prescriptions for corticosteroids.101 The early-morning circadian rise of endogenous T363 appears to drive early-morning cortisol release that is critical to health.101,102 Note that there is substantial knowledge on the benefits of treating hypothyroidism and low metabolism due to isolated low T3 levels. As discussed above, T4-treated hypothyroid patients often have low T3 levels, and they benefit remarkably from combination T3/T4 that is adapted individually to resolve their symptoms.103,104 Some patients even experience periods of debilitating exhaustion where they are “stuck” in a state of low metabolism.
Plasma T3 is often reduced and rT3 is elevated - indicating LT3S - and T3 therapy is required to recover. Patients report that they increase T3 intake for several weeks, often while simultaneously reducing or stopping T4 intake.105,106 Plasma T3 increases to high-normal or even supraphysiologic levels, but the patient’s metabolism remains low or low-normal. After a typical period of 8-12 weeks, metabolism rises rapidly to normal (within hours or days). The patient then returns to their normal T3 and T4 intake, repeating the same procedure if LT3S occurs again. The treatment efficacy likely stems from the ability of the added T3 to raise intracellular T3 levels, increasing cellular metabolism and transport (which is active, requiring ATP) and tipping T4 conversion toward T3 and away from rT3.46,47 Some patients cannot tolerate any T4 and require lifelong T3 monotherapy. Unfortunately, T4-induced LT3S is an unstable and dangerous aspect of T4 therapy that is not widely recognized or treated.
Unusually low metabolism without diagnosed low T3 or hypothyroidism has been observed and treated successfully with T3 for decades. For example, in 1957, Dr Joseph Morton reported a state of low metabolism that responded to T3 treatment but not to natural-desiccated thyroid hormone, which contains T4 and T3 in a ratio of 4:1 nominally.107 More recently, Dr Dennis Wilson pioneered the use of sustained-release T3 for normalizing depressed metabolism and basal temperature in symptomatic but chemically “euthyroid” subjects.108 His method has been successful more broadly.109 These observations all point to low intracellular T3 as the underlying issue causing low metabolism, regardless of whether it is reflected in low plasma T3 levels as in classic LT3S.46,47
Further studies are needed on thyroid-hormone treatment of LT3S to demonstrate efficacy and raise awareness within the broad medical community.
Thyroid patients are seldom included in studies of LT3S.110 The few LT3S trials to date have had mixed results, leading to lack of consensus on treatment and leaving doctors and patients to use their clinical judgement. (Mechanistically, T4 is expected to be counterproductive while direct T3 is expected to be beneficial,111 and one group recommends T3 over T4 treatment.112) Some doctors think LT3S is a protective adaptation that, for example, increases animal survival in the wild by reducing metabolism and caloric requirements in times of scarcity.
However, this theory is undermined by the absence of evidence supporting a beneficial role of LT3S in survival and by substantial evidence showing that LT3S patients can only survive and recover in modern hospitals.16 Oxidative stress (OS) and inflammation are also under control of thyroid hormones, and increased oxidative stress is observed in hypothyroidism113 and LT3S.114,115 According to Mancini et al., “The presence of OS indexes in NTIS supports the hypothesis that it represents a condition of hypothyroidism at the tissue level and not only an adaptive mechanism to diseases.”114 Most likely, LT3S is an “imperfect” adaptation that becomes pathological when severe or in certain genotypes.
Low T3 In Eating Disorders
Calorie reduction from any cause generally results in decreased circulating T3. In eating disorders, T3 reduction depends on the disorder subtype, where it appears that more severe calorie restriction causes the greatest decrease in T3.116,117
As reviewed by Troisi et al. in a stufy of 50 women:117 anorexia nervosa (AN) involves restricted eating (and sometimes purging) due to fear of weight gain and usually results in weight loss. Bulimia nervosa (BN) typically involves periods of uncontrollable eating followed by selfinduced vomiting, dieting or intense exercise to prevent weight gain; BN patients usually remain at normal body weight. Binge-eating disorder (BED) also involves compulsive eating, but without compensatory behavior of BN; therefore, BED patients are usually overweight or obese. In the study, free T3 and BMI were lowest in the AN group. The BN and BED groups also had lower free T3 than controls, but BMI was higher than controls.
The patient in the case study presented in this paper had a pattern of restricted eating, weight loss, and change of circulating T3 similar to the AN subtype but with a different etiology as it was precipitated by GI distress and nausea.
Gut Microbiota, Micronutrients, and Autoimmunity
Gut dysbiosis and thyroid disorders are intertwined through complex interactions among the gut microbiota, micronutrient levels and uptake, thyroid hormone formation and function, absorption of oral thyroid hormones, and autoimmunity.118-120 Gut microbiota influence the uptake of minerals that support thyroid function, some of which modulate the microbiota composition. Gut dysbiosis can upset the balance of key minerals - notably iodine, selenium, iron and zinc - locally and in the circulation:118-120
- Iodine is an essential constituent of thyroid hormones, and it also has extrathyroidal actions as an antioxidant, differentiator, apoptotic, immunomodulator, and in supporting cellular processing of thyroid hormones.121-124 Iodine uptake, degradation, and enterohepatic cycling are regulated by gut microbiota and influence thyroid hormone levels.
- Selenium is integral to several proteins involved in thyroid metabolism, including the deiodinase (selenodeiodinase) enzymes D1, D2 and D3 that activate and deactivate thyroid hormones, and the glutathione peroxidase family of antioxidants.125-127 Glutathione peroxidase reduces and neutralizes hydrogen peroxide - which is formed by thyroperoxidase (TPO) during thyroid-hormone synthesis - protecting the thyroid gland from oxidative damage that can trigger and promote Hashimoto’s thyroiditis, an autoimmune attack on TPO itself.125-127 Selenium increases microbial diversity, favoring in particular the growth of probiotic (beneficial) Bifidobacterium adolescentis. Microbes in the colon help metabolize selenium for absorption by the host.
- Iron is needed for thyroid-hormone biosynthesis as a key constituent of the active center of TPO. Iron balance is important in the gut, where it can change the levels of healthy and detrimental microbes and even promote bacterial overgrowth. Microbiota in the colon can increase iron availability and absorption by producing short-chain fatty acids (SCFAs) that reduce pH.
- Zinc increases the conversion of T4 to T3 by enhancing the activity of D2. Zinc deficiency has been shown to reduce circulating T4 and T3 levels. There is evidence that zinc modulates the microbiota composition, and in humans, zinc supplementation can help prevent diarrhea by “inhibiting the growth of pathogenic Escherichia coli, and promoting the growth of probiotic strains such as Lactobacillus spp.”118
These minerals are often deficient in hypothyroidism, and deficiency of these minerals renders patients hypothyroid, independent of circulating thyroid-hormone levels; supplementation is effective and necessary for recovery.118-120,123,124,128,129 Iodine should be taken with selenium, with dose adapted individually to the patient to maximize benefits while minimizing oxidative stress and the potential for triggering or exacerbating hashimoto’s autoimmunity.123-127 Lugol’s iodine4 is the most popular form, and doctors and patients have reported highest success using it with companion nutrients that include selenium and magnesium.106,126,130,131 Vitamin D is also often low in hypothyroid patients and may be a factor in autoimmune disease, particularly Hashimoto’s thyroiditis.119,132
Intestinal bacteria also play a role in the synthesis of many vitamins, including vitamin K, folate, vitamins B2, B3, B5, B6, B7, and B12. Gut microbes also have deiodinase activity, helping to convert T4 to T3, and they can also help store T3 and reduce fluctuations in circulating thyroid hormone levels. Synbiotic supplementation (a combination of pro- and pre-biotics) was recently shown to benefit hypothyroid patients by significantly increasing free T3 and reducing fatigue, T4 dose, and TSH.118,119
The gut microbiota have significant roles in immune function and autoimmunity. SCFAs produced by gut microbes can be used by enterocytes for energy and work together with T3 to maintain the epithelium and integrity of intercellular tight junctions (TJs).118,133 Altered microbiota can promote autoimmunity by compromising TJs, leading to leaky gut, and by several other proposed mechanisms.118 Microbial antigens can translocate across the intestinal wall and generate autoantibodies that cross-react with appetite-regulating hormones and neurotransmitters.134 SCFAs and many other microbial metabolites also have important metabolic and signaling functions, modulating the integrity of the blood-brain barrier (BBB), brain function, and other aspects of host homeostasis.135 Starvation and weight loss in AN may compromise the BBB and promote the production of pro-inflammatory cytokines. It is also thought that AN and BN can be triggered by a weakened BBB allowing access of cross-reactive autoantibodies to brain centers.134,135 Bacterial overgrowth can also be an issue in hypothyroidism. Normal levels of gastric acid are an effective barrier to overgrowth of the microbiota,77 but in hypothyroidism, weak stomach acid may lead to bacterial overgrowth and malabsorption of oral T4, as explained by FröHlich et al118:
Hypothyroid patients, particularly those with Hashimoto’s thyroiditis, sometimes struggle with dyspepsia, acid reflux, bloating, incomplete digestion, SIBO, poor absorption of iron and vitamin B12, and other consequences of weak stomach acid or elevated pH (which is likely due to subnormal gastrin136). Doctors and patients have many
approaches to improving digestion, including bitter herbs, digestive enzymes, and dilute vinegar (for the acidity). Betaine HCl is a very popular and effective oral supplement that can quickly lower pH.137,138
Appetite-Regulating Hormones
Many hormones that control appetite, hunger and motility are produced in the GI tract and are influenced by the gut microbiota.139,140 These hormones are broadly divided into appetite stimulators, such as ghrelin, and satiety stimulators, such as leptin, glucagon-like peptide 1 (GLP-1), peptide tyrosine- tyrosine (PYY), and cholecystokinin (CCK). Many of these hormones have overlapping actions, which is indicative of biological redundancy.141 Leptin reflects the body’s energy stores and is secreted mainly from the white adipose tissue, but also from the stomach and intestines, which contain leptin receptors.139 Release of these hormones is influenced substantially by the metabolites of gut microbes, which vary among eating-disorder subtypes.139,142
Thyroid hormones have important roles in appetite regulation and energy balance, alone and in concert with other hormones, particularly leptin.33,143,144 Notably, T3 acts directly on the hypothalamus to stimulate appetite and food intake31,32 at physiological levels, and the effects are rapid and independent of energy expenditure, as described by Kong et al32:
These observations suggest a powerful role for oral T3 therapy in raising appetite and food intake in ED patients at moderate doses that avoid overstimulating metabolism.
LT3S Cycle In Eating Disorders
LT3S in eating disorders is a self-reinforcing cycle of dysfunction, as shown in Figure 1. Lowcalorie intake, especially if prolonged, induces low metabolism via LT3S, which lowers GI motility, causing chronic constipation and associated feelings of fullness that inhibit eating and sustain the disorder. The various triggers, discussed in more detail above, can help sustain the process, including pre-existing hypothyroidism and T4 therapy that can compromise GI motility.29,77,145 Standard treatment involves various interventions to increasing food intake with the goal of reversing lt3s and restoring normal hunger and eating patterns. For children, the main approach is to have parents plan meals and monitor the child’s food intake while encouraging them to eat more and tolerate the discomfort. Sometimes, feeding tubes are used in the hospital to directly increase calorie intake, and as a tactic to scare the patient into compliance. Gastrointestinal pro-kinetic drugs such as erythromycin are also an option. Family-based therapy helps by getting the whole family to act as a team in the treatment process. Eating eventually returns to normal in many patients. (Recurrence due to ongoing psychological factors, such as in AN and bulimia, are outside the scope of this article.) However, evidence indicates that long-term caloric restriction causes irreversible reduction of T4-to-T3 conversion and cellular transport, disposing patients to recurrence of the eating disorder.38,46,47,146,147 Note that hormonal intervention with T3 can reverse or alleviate LT3S, and may be necessary in difficult eating disorder cases. In some patients, metabolism rises immediately with increasing T3 intake. Other patients are less fortunate and get into the irreversible, low-metabolic LT3S state described above.5
Pre-existing autoimmune hypothyroidism adds a further level of complexity. Hashimoto’s autoimmune thyroiditis causes psychiatric issues in itself151,152 and can complicate the presentation and treatment of AN, as described by Kızılkan et al.153 Hashimoto’s can also present with primary adrenal insufficiency in autoimmune polyendocrine syndrome type II (APS II or Schmidt Syndrome), in which adrenal crisis can be precipitated by levothyroxine use.154 In combination with the induced hypothyroidism of LT3S, these issues could potentially exacerbate any of the mental, psychological, and physical maladies of hypothyroidism, some of which are described earlier.
In the case presented here, prolonged gut dysbiosis and nausea triggered reduced eating to initiate the LT3S cycle; however, the patient was at risk due to pre-existing hypothyroidism, T4 usage, and history of constipation.
Resolution of signs and symptoms must be the main goal of hormone treatment; laboratory tests are unreliable. TSH is often low in LT3S and therefore an inaccurate marker of hypothyroidism and low metabolism.16,47 As discussed above, TSH also appears to be unreliable for diagnosing and monitoring hypothyroidism in general: TSH is controlled by T3 level in the pituitary gland, which converts T4 to T3 more efficiently and reliably than other tissues of the body in health and in illness.46,47,54,71,155 T3 can be low in the blood and other tissues while TSH is also low due to efficient T4-to-T3 conversion in the pituitary. Quoting the recent consensus document from the American, British, and European Thyroid Associations54: “Thus, while increasing the LT4 replacement dose to treat hypothyroidism, normalization of serum TSH levels will occur before full normalization of serum T3 levels.” This also helps explain why TSH is usually low or suppressed when thyroid-hormone dose is raised sufficiently to resolve signs and symptoms, as reported by prominent doctors over the decades.50,53,156 And finally, while we used T3 to treat the patient in this study, administering TSH or TRH (thyrotropin releasing hormone) are other potential options in LT3S.16
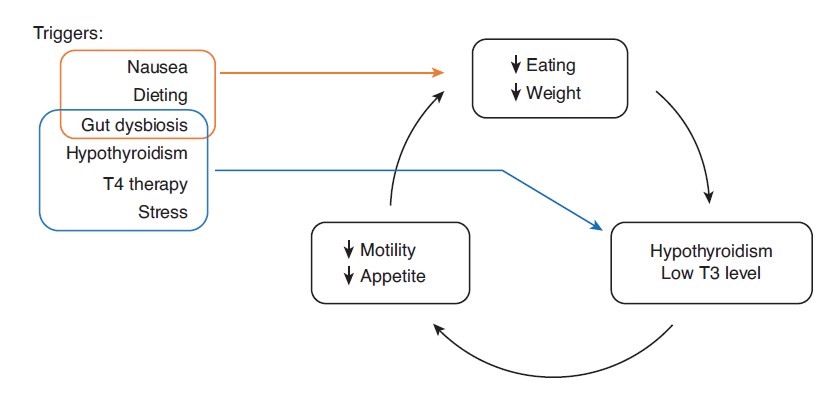
Case Presentation
From birth, the patient was healthy and had regular immunizations. Age 2-4 she had childhood ear infections at about the same frequency or slightly higher than other kids, and she was treated with antibiotics. In second grade (2013), she had a difficult period with significant constipation. This caused severely restricted eating for a few weeks, and she lost weight and withdrew socially, which affected some of her relationships negatively. Her pediatrician ordered x-rays that confirmed constipation and recommended Miralax (polyethylene glycol 3350), which was effective at relieving the constipation, while drinking more water and eating more dietary fiber was not.
Her father observed that she continued to have some degree of ongoing constipation following second grade. Prior to this, she usually ate breakfast every day. However, afterwards, she often skipped breakfast and, when questioned, described lack of hunger and constipation. She also became noticeably less affectionate and engaged, and less interested in playing with her parents and younger brother.
During the 2013 to 2017 period leading up to the eating disorder, she also had difficulty concentrating in class (described as spaciness or “brain fog”) and auditory distraction. The Children’s Health Council (Palo Alto, CA) assessed her and reported a disconnect between intelligence and achievement - that is, the inattention was interfering with achieving to her above-average intelligence level.
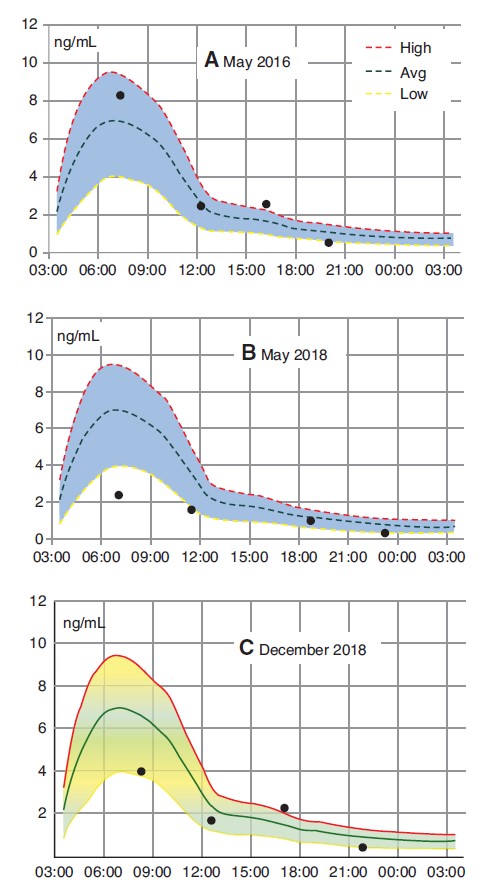
Her father began suspecting hypothyroidism in 2013 due to these and other issues that formed a cluster of signs and symptoms: ongoing constipation, inattention and difficulty concentrating in school, dry skin, weak, delaminating fingernails, dark circles under the eyes, chronic insomnia, and family history of both parents. Her TSH rose to 7 mIU/L at age 10 in 2016, and she tested positive for TPO and thyroglobulin (TG) antibodies, indicating Hashimoto’s autoimmune thyroiditis. Salivary cortisol (ZRT Laboratory) was within the normal range in May 2016, as shown in Figure 2. she began thyroid-hormone treatment at an integrative medicine practice soon thereafter.
Initial treatment with 25 μg T4 (SR) degraded her condition: constipation and inattention increased while energy decreased, and blood testing showed increased levels of rT3. Symptoms abated with combination SR T3/T4 therapy, which was continued thereafter. After the Hashimoto’s diagnosis, TPO antibodies remained in the 200-IU/mL range, while TG antibodies have only risen above zero in one or two blood tests.
She stopped eating gluten in early 2017 (due to the crossover with autoimmunity) and has been diligent. There was no change of TPO and TG antibodies and her parents did not notice any change of mood. Upon taking selenium, TPO and TG antibody levels both decreased slightly, but any long-term effects have been unclear, compounded by inconsistent selenium intake.
Her mental focus, engagement, and sleep patterns are affected greatly by hypothyroidism and thyroid hormone treatment. From the beginning of treatment, her dose had to be increased periodically at least four times over 2 years as she grew.
Insomnia was always the lead symptom, and all symptoms abated after dose increases.
Family History
The mother was diagnosed with hypothyroidism (elevated TSH) in her mid-teens after she reported tiredness and increased need for sleep from 8 to 12 hours per night. Treatment proceeded with T4, to which she responded with increased energy and weight reduction within normal range. Blood tests in 2014 showed high rT3 when on T4 monotherapy.
She then began taking compounded SR T4/T3, and rT3 decreased to within the reference range.
The father experienced the clinical features of hypothyroidism for 1-2 years and, upon treatment, had a severe adverse response to rapid-release T4, suffering extreme fatigue, brain fog, muscle cramps, achy joints, and heart palpitations. He made a full recovery with SR T3/T4 and Lugol’s iodine (brand Iodoral or LugoTab) taken with companion nutrients (selenium, magnesium, niacin, and riboflavin).106,126,130,131 The patient’s paternal grandmother was diagnosed with Hashimoto’s autoimmune thyroiditis in her 50s or 60s but only began treatment with thyroid hormones at age 90. She had a mild ischemic stroke around age 70 years due to atrial fibrillation and was treated with digoxin and coumadin thereafter.
She passed away at age 94 due to internal bleeding from coumadin poisoning.
Onset Of Illness
The patient began complaining of nausea in late November 2017 when she was just completing the second of two rounds of antibiotics for strep throat. Her nausea persisted, causing food intake to decrease significantly for weeks. She also began experiencing variable levels of energy and constipation.
At 6-8 weeks, she began experiencing increasing levels of anxiety that culminated in school refusal. Old and new signs and symptoms appeared including insomnia, constant scratchy sore throat, delaminating fingernails, feeling of restless legs, cold-like symptoms, dizziness, blurred vision, dark, shiny circles under eyes, and jaundice (yellow tint to whites of her eyes). She also began avoiding any food that she thought could possibly worsen the GI distress and was diagnosed with ARFID (avoidant/ restrictive food intake disorder) that lasted over 1 year.
She began missing school more and more frequently in January 2018 due to nausea and gut pain, which would come on after meals and randomly.
Her pediatrician prescribed ranitidine (Zantac) to reduce stomach acid, but it did not help with symptoms. She also had stressful emotional experiences when a foot injury and conflict with peers caused her to drop out of ballet class, which had been central to her life for about 8 years.
SIBO was diagnosed in late January 2018 by breath test. Since adequate stomach acid (low pH) helps inhibit bacterial overgrowth (as discussed above) we discontinued Zantac and switched to Betaine HCl; nausea after meals was immediately reduced or eliminated. She began SIBO treatment with botanicals157 and then rifaximin (Xifaxan) and nystatin for 10 days.158 The nausea and gut pain improved clearly after 2 weeks but did not fully resolve. She only went to school partial days after this time. in march, she was withdrawn from friends and family, spending most of her time in bed feeling exhausted with stomach pain, falling asleep late (12-1 am) with insomnia, and waking late (10-11 am). A psychiatrist diagnosed generalized anxiety disorder and depression and prescribed a selective serotonin reuptake inhibitor (SSRI) and benzodiazepine, but the patient was non-compliant.
Thyroid-Hormone Treatment
In early March, laboratory blood tests (Quest Diagnostics; see Table 1) showed low levels of thyroid hormones: free T3 (fT3) was below normal while free T4 (fT4) was low-normal. In late March, saliva tests (ZRT Labs; see Figure 2) showed low levels of cortisol, indicating hypothalamic- pituitary-adrenal (HPA) axis dysfunction. (Note that she had normal cortisol in 2016, also shown in Figure 2.)
Doctors began adapting her thyroid dose in late March. Table 2 indicates progression of the clinical features between time periods of approximately 3 months, while Table 1 shows laboratory test results. Figure 3 shows the treatment progression in graphical form.
March-May 2018: T3 dose increase, improvement of demeanor and food intake
Thyroid-hormone treatment commenced at an integrative medicine facility in late March, with T3 raised carefully in increments of 1 μg every 3 days, using a sustained-released formulation in capsules made by Koshland compounding pharmacy (San Francisco, CA). Per the doctor’s instruction, the father checked daily for signs of clinical hyperthyroidism, including elevated pulse rate; none were observed. Within 10 days the father observed positive changes: the patient became brighter, funnier, and more affectionate, energetic and engaged, her sleep patterns improved (sleep ≈10 pm, wake ≈8:30 am), and she attended school briefly one day (the first time in weeks). Constipation and nausea diminished.
She began a prescription of hydrocortisone 2.5 mg; however, it was discontinued after 5 days as it did not seem to help with symptoms, and she complained of increased irritability. Pregnenolone 12.5 mg also had no effect on symptoms and was discontinued after 1-2 months. Improvement in her condition correlated only with increasing T3 intake during this time.
In mid-April, the family hired a personal therapist with training and experience in adolescent school refusal. She had some initial, short-lived success getting the patient to go out to local stores but not with school refusal.
In early May, her father drove her with a good friend to a national park for a 1-week school trip.
She socialized and interacted well with others in the campground but, citing anxiety, did not go on group outings such as hikes and ate all meals with her father away from others. She complained of constipation, nausea, and feeling full upon eating or drinking very small amounts.
After the trip, her parents took her to the emergency room (ER) to check weight loss and vital signs.
Weight was 37.7 kg (83 lbs), and vital signs were normal - blood pressure 105/69 mmHg, pulse 65- 70 bpm, temperature 36.7 - 36.9°C (981 - 98.5°F), electrolytes normal - except for high orthostatic pulse change. The staff psychiatrist met with the patient and said that she was not depressed. This fit with the patient’s recent social behavior, which was limited by anxiety rather than depression.
She began taking Miralax daily, and the constipation abated along with the intensity of nausea.
Morning hunger increased and she began eating breakfast, lunch, and dinner each day. She was still disinterested in meat and fish, which she had enjoyed prior to the illness. Weight had increased to 39.6 kg (87 lbs) at a laboratory blood draw a few days after the ER visit (height 1.63 m/64 inches).
She began seeing friends more often and reported feeling less overwhelmed. However, she still felt anxiety in group situations and would only eat with her immediate family, and she was still eating small meals.
Many thyroid-related symptoms improved or resolved after increasing T3 intake between March and May 2018, including mental focus, insomnia, tiredness, depression, and dry skin. Blood tests mid-
May (Table 1) showed low-Normal Ft3 And Ft4, and improved liver function (bilirubin and ALT).
The family hired a new psychiatrist with expertise in PANS and PANDAS (Pediatric Acute-onset Neuropsychiatric Syndrome, and Pediatric Acute onset
Neuropsychiatric disorders associated with Streptococcal infections) because the illness had started after a bout of strep throat, which is a typical trigger of neuroinflammation that underlies these conditions. However, there was no other objective or subjective evidence to support a PANS diagnosis: blood markers such as elevated C-reactive protein were negative. Symptoms and progression did not fit the criteria for PANS, in which neurological changes occur within 48 hours of strep throat and cause rapid changes of personality such as obsessive-compulsive disorder (OCD) and degradation of handwriting. We held off on PANS-specific treatment at this time.
June-August 2018: hospitalization reduced thyroid dose, degraded health and demeanor
The patient entered a hospital eating-disorders unit due to weight loss and unstable vital signs (high orthostatic pulse change). Food intake decreased for 2 days and then increased through the rest of the 18-day stay with concomitant decrease of nausea and discomfort (feeling of fullness). The attending physician declined to accept or consider the onepage patient health history prepared by the father, which included the recent improvements observed with small T3 dose increases. The physician was also uncomfortable with the patient taking T3 and lowered the patient’s T3 and T4 intake a few days before discharge.
Immediately after discharge, the patient was able to socialize with friends outside of home, indicating some improvement in anxiety, and she continued to eat large meals for a few days.
Around 10 days after discharge nausea increased, and food intake, mood, and sleep patterns degraded continually. One month after discharge, we raised her T3 and T4 dose slightly and her condition stopped degrading but did not improve.
The hospital did not follow up on the change of thyroid-hormone dose to see how it affected the patient. Six weeks after discharge, she started 12.5 mg of sertraline SSRI (considered to be half of an effective dose) following the advice of the psychiatrist, pediatrician, and doctors of the eating-disorders program. She began complaining of nausea after 24 hours and food intake decreased the next few days. An increased dose of 25 mg sertraline at day 3 resulted in increased nausea such that the patient could barely eat. We discontinued sertraline on day 5; nausea abated and food intake rebounded in 36-48 hours.
August-December 2018: Increased Thyroid dose and improved mood
In mid-August, we began increasing T3 by 2 μg every 14 days. Mood and energy began improving after 4 days and she began to socialize with friends in the neighborhood.
Two weeks after the first dose increase, she began attending school for brief (10-20 minute) periods in a private room with a tutor and away from the other school kids.
At the end of August, she was taking the same thyroid-hormone dose as she had been taking at the time she was admitted to the hospital.
By Mid-September, her energy and mood had improved significantly, and she was attending school for 2-3 hours each day in the private room with a tutor. She still experienced nausea that interfered with eating; Betaine HCl was helping substantially and allowed her to eat more. Her taste for meat returned and she began eating ham and beef.
In early October, the 2-μg T3 dose increase had diminishing immediate effects on her energy (on the time scale of 1-2 weeks). Orthostatic vital signs were normal. We decided to hold the thyroidhormone dose constant and allow time for the T3 to take full effect while doctors of the extended group tried different treatments.
Salivary cortisol in late December is shown in Figure 2. Cortisol was low but in-range most of the day - an improvement from March.
October 2019 To January 2020: Failure of conventional treatments and rehospitalization
In mid-October, to address potential neuroinflammation from PANS, the psychiatrist started a 5-day prednisone burst to suppress the patient’s immune system, with the intent of resetting it. She reported increased anxiety and depressive symptoms during the prednisone course and recovered within about 5 days after completion, with no noticeable long-term changes in health or wellbeing. In early
November, she began taking the antihistamine cyproheptadine (Periactin), prescribed by a gastroenterologist with the intent of reducing nausea by calming the gut-brain connection. Over the next few days, she became increasingly tired, dizzy, and depressed, and the parents and gastroenterologist discontinued the medication.
In early December, two family members (but not the patient) came down with a stomach illness.
The patient began avoiding food and utensils in the house for fear of catching the illness, and her weight began to decrease.
At the end of December, the patient began taking 2.5 mg escitalopram SSRI (Lexapro) from the psychiatrist, who intended to increase to a therapeutic dose over time. Higher doses caused an immediate increase in nausea and reduced eating. she continued with 2.5-mg dose after January. (The patient continued this dose until August 2020 and then stopped with no changes to mood or wellbeing.) Avoidant eating and weight loss continued, and the patient was re-admitted to the hospital in early January for 5 days. The hospital doctors understood that the patient had lost weight due to avoidant eating, reduced eating because of nervousness around catching the stomach illness from the family.
However, they lowered her thyroid-hormone (T3) dose again, stating the patient had low-normal TSH that could have caused weight loss. One day later, the patient complained of feeling tired and low.
Upon leaving the hospital, the prior T3 dose was reinstated, and the patient recovered energy and continued to gain weight.
February-June 2019: Patient Attends Classes at a new school
The patient went on a family trip to the snow country and enjoyed it, to her surprise. The family also hired a new therapist who began to have success with exposure therapy. She began attended class in a new, private school with help of the new therapist.
Her mood and social interactions improved further upon increasing T4 intake over the next few months, and she started menses.
October-November 2019: Increased socialization, wellbeing, weight, and height
The patient became increasingly social and emotionally resilient. Her weight and height continued to increase through November, at which point she stopped regular check-in visits to the eating-disorders clinic.
Discussion And Conclusions
The patient history prior to the eating disorder included weak fingernails, dry skin, difficulty concentrating, insomnia, constipation, and ensuing periods of reduced calorie intake that suggest hypothyroidism began at age 7 in 2012. However, it took 4 years for her TSH to rise out of range and indicate biochemical hypothyroidism. When treatment was initiated in 2016, she responded poorly to T4 monotherapy - with decreased energy and increased rT3 - and well to combination, SR T3 and T4 at a ratio of about 1:10 with improvements in insomnia, energy, attention, skin quality, and fingernail strength. (She also learned to take her medications regularly and remained diligent with the changes throughout the eating disorder, as noted by her father.) Therefore, leading up to the eating disorder, she would have been considered a “poor responder” or “treatment resistant” and had a relatively narrow therapeutic window for thyroid-hormone treatment.
Various factors could have contributed to the narrow therapeutic window. Extended periods of restricted eating - such as the 4-year period ending in 2016 - could have induced permanent resistance.46,47
Her father’s severe negative response to T4 monotherapy suggests a contribution from genetics. As discussed in the Introduction, she may have had an inherently strong, individual protective response to excess thyroid hormone concentration upon absorbing an unphysiologic oral dose: the body overreacts to prevent hypermetabolism (thyrotoxicosis), resulting instead in depressed metabolism. Hashimoto’s autoimmunity complicates the case further. All of these would factor into her clinical thyroid status and modify her response to thyroid-hormone treatment as her food intake decreased and stress increased leading to the eating disorder. It is also possible that the patient was undermedicated leading into the eating disorder, given that her thyroid-hormone dose had to be adjusted upward every few months as she grew.
Her need for T3 and T4 increased substantially during the eating disorder, when she also grew rapidly and went through puberty. Figure 4 shows her intake of T3 as a fraction of T4. During the main course of the eating disorder, T3 needed to be increased relative to T4. The T3/T4 ratio was substantially above 25% between March 2018 and May 2019. After May 2019, the T3/T4 ratio settled to around 25%.
However, the total dose continued to increase as she grew and became more active, as shown in Figure 3.
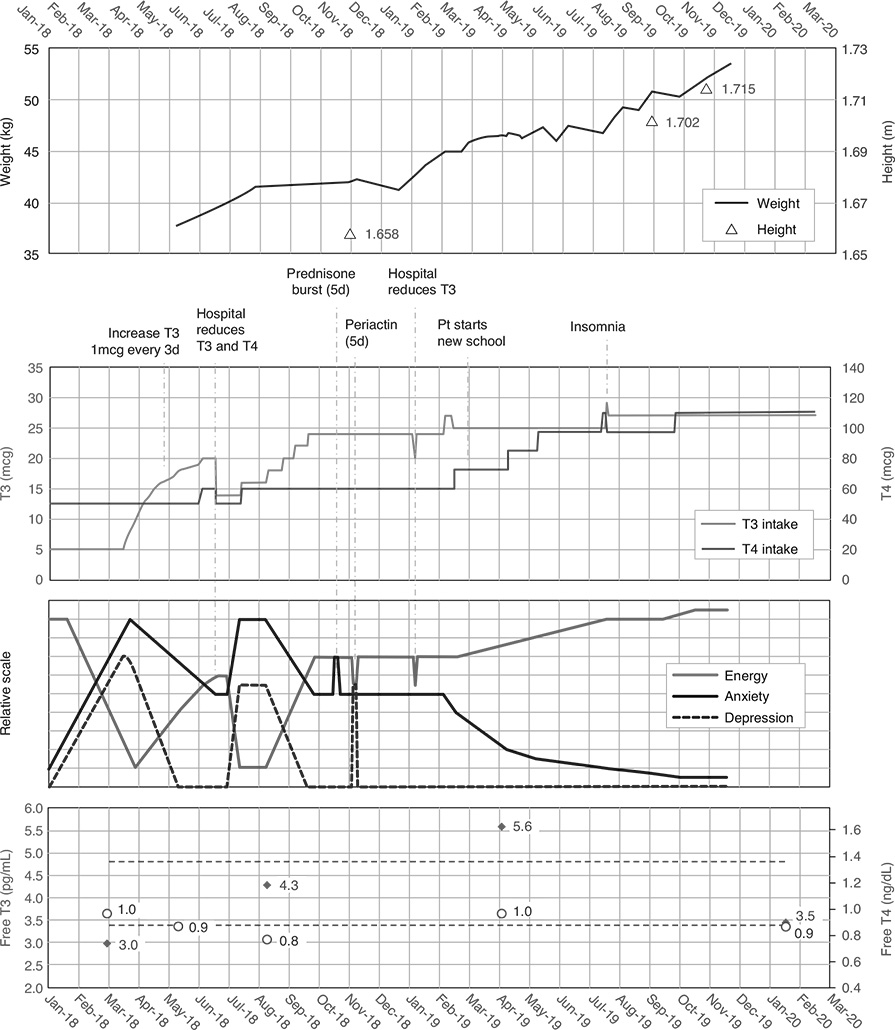
(The dotted line in Figure 4 is 25% T3, which is equivalent to Thyroid USP - desiccated thyroid extract, or DTE - which many doctors and patients still use routinely to treat hypothyroidism.) The literature has ongoing discussions around mechanisms leading to the low T3 level seen in LT3S, including the timing and order of changes that occur in deiodinases and other hormones.15 Elevated glucocorticoids (cortisol) and pro-inflammatory cytokines were implicated initially, but newer evidence points to the cycle beginning with changes of T3 and T4 levels themselves that then cause changes in the deiodinase enzymes.15 The patient in this case had low - not elevated - saliva cortisol (Figure 2) and low T3 in March 2018, early in the eating disorder. She responded neutrally or poorly to low-dose hydrocortisone (2.5 mg, SR), which was discontinued after 5 days. In contrast, she responded well to T3 and T3/T4 therapy, with cortisol rising to low-normal in December 2018.
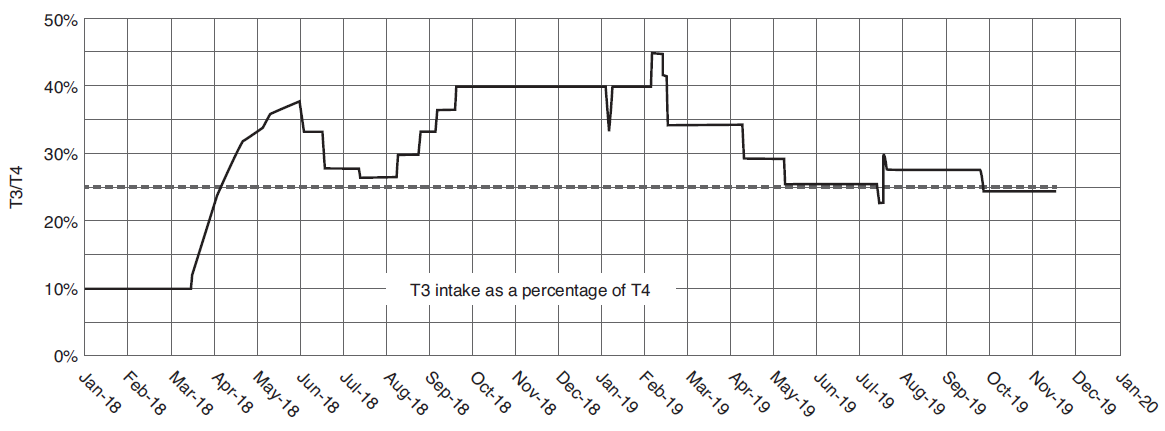
This suggests that the thyroid dysfunction precipitated the low cortisol rather than vice versa.
Exposure therapy helped the patient reintegrate academically and socially, but only after she had recovered significant mental and physical function with thyroid-hormone therapy. Earlier in the treatment, nurturing counselors and well-trained therapists had little success.
Update On The Patient, November 2021
The patient’s health and wellbeing have improved continually with ongoing careful thyroid treatment.
Her thyroid dose increased to 30 μg T3 and 127 μg T4 (all in SR form) and has been steady over the last year along with her height of 1.74 m (68.5 inches) and weight of 58.1 kg (128 lbs). She discontinued taking escitalopram SSRI (2.5-mg dose) in August 2020 with no changes to mood or wellbeing. Since going through puberty, her iron levels have been low along with vitamin D, folate and zinc, and supplementation has been important.128,129,159-161 She began attending a mainstream public high school this year as a junior and has developed a large circle of friends. Tennis is her main sport.
The T3 and T4 dose adjustments correlated strongly with improvements to her health and wellbeing, including improvements in constipation, food intake, anxiety, depression, outgoingness, and sleep patterns, suggesting the myriad, powerful effects of thyroid hormones and a likely causal link. That thyroidhormone treatment was successful when multiple conventional treatments failed underscores the need for further ED/thyroid studies. Unfortunately, the patient’s progress may also have been constrained by well-intentioned doctors who did not follow sound medical practice, such as accepting (or taking) a proper medical history and supporting fundamental (thyroid-hormone) treatment that was underway and showing clear efficacy. These actions are not unique in thyroid therapy and reflect the state of the field as discussed earlier. This presents a substantial problem for parents of children with eating disorders and for patients themselves. In most cases, they will not be given complete information about the fundamentals of the disorder or the option for thyroid-hormone treatment that could be beneficial or even lifesaving.
Parents must recognize this and plan accordingly by finding experienced doctors. Parents know their children best and are their best advocates. This case illustrates the efficacy of adapting thyroid-hormone treatment to the patient in different circumstances of life to optimize and maintain health. It also demonstrates the need for unbiased observation with “high degree of suspicion” when diagnosing and treating hypothyroidism.
Acknowledgements
The authors thank Rudolf Hoermann for insights in hormonal-control mechanisms and APS II.
Availability Of Data And Materials
All data generated or analyzed during this study are included in this published article.
Competing Interests
The authors declare that they have no competing interests.
Funding
Not applicable.
Consent For Publication
Written informed consent was obtained from the patient for publication of this case report. A copy of the written consent is available for review by the Editor in Chief of this journal.
Authors’ Contributions
Both authors contributed to the body of work and read and approved the final manuscript. SW wrote the initial draft with a review of mechanisms and biochemical pathways. RS and SW expanded, integrated, and reorganized the concepts around etiology, treatment, activity in the field. and historical context.1000,2000-3000,3100
References
- Brent GA. Mechanisms of thyroid hormone action. J Clin Invest. 2012;122(9):3035–43. doi:10.1172/JCI60047.
- Montesinos M del M, Pellizas CG. Thyroid hormone action on innate immunity. Front Endocrinol (Lausanne). 2019;10:1–9. doi:10.3389/fendo.2019.00350.
- Faustino LC, Ortiga-Carvalho TM. Thyroid hormone role on cerebellar development and maintenance: a perspective based on transgenic mouse models. Front Endocrinol. 2014;5:75. doi:10.3389/fendo.2014.00075.
- Schroeder A, L Privalsky M. Thyroid hormones, T3 and T4, in the brain. Front Endocrinol. 2014;5:40. doi:10.3389/fendo.2014.00040.
- Heinrich T, Grahm G. Hypothyroidism presenting as psychosis: myxedema madness revisited. Prim Care Companion J Clin Psychiatry. 2003;5(6):260–6. doi:10.4088/pcc.v05n0603.
- Hall RCW. Psychiatric effects of thyroid hormone disturbance: psychosomatic illness review: No. 5 in a series. Psychosomatics. 1983;24(1):7–18. doi:10.1016/S0033-3182(83)73255-X.
- Constant EL, Adam S, Seron X, et al. Anxiety and depression, attention, and executive functions in hypothyroidism. J Int Neuropsychol Soc. 2005;11(5):535–44. doi:10.10170S1355617705050642.
- Shames R, Shames K, Shames GG. Thyroid Mind Power: The Proven Cure for Hormone-Related Depression, Anxiety, and Memory Loss. Rodale Books; 2011.
- Premachandra BN, Kabir MA, Williams IK. Low T3 syndrome in psychiatric depression. J Endocrinol Invest. 2006;29(6):568–72. doi:10.1007/BF03344150.
- Razvi S, Jabbar A, Pingitore A, et al. Thyroid hormones and cardiovascular function and diseases. J Am Coll Cardiol. 2018;71(16):1781–96. doi:10.1016/j. jacc.2018.02.045.
- Zhang Y, Dedkov EI, Teplitsky D, et al. Both hypothyroidism and hyperthyroidism increase atrial fibrillation inducibility in rats. Circ Arrhythm Electrophysiol. 2013;6(5):952–9. doi:10.1161/CIRCEP.113.000502.
- Abdel-Moneim A, Gaber AM, Gouda S, et al. Relationship of thyroid dysfunction with cardiovascular diseases: updated review on heart failure progression. Hormones. 2020;19(3):301–9. doi:10.1007/s42000-020-00208-8.
- Kannan L, Kotus-Bart J, Amanullah A. Prevalence of cardiac arrhythmias in hypothyroid and euthyroid patients. Horm Metab Res. 2017;49:430–3. doi:10.1055/s-0043-105275.
- Gogakos AI, Duncan Bassett JH, Williams GR. Thyroid and bone. Arch Biochem Biophys. 2010;503(1):129–36. doi:10.1016/j.abb.2010.06.021.
- Warner MH, Beckett GJ. Mechanisms behind the nonthyroidal illness syndrome: an update. J Endocrinol. 2010;205(1):1–13. doi:10.1677/JOE-09-0412.
- DeGroot LJ. The non-thyroidal illness syndrome. In: Feingold KR, Anawalt B, Boyce A, et al., eds. Endotext [Internet] https://Www.Ncbi.Nlm.Nih.Gov/Books/NBK285570/. MDText. com, Inc. 2015. doi: https://www.ncbi.nlm.nih.gov/books/NBK285570/.
- Bianco AC, Dumitrescu A, Gereben B, et al. Paradigms of dynamic control of thyroid hormone signaling. Endocr Rev. 2019;40(4):1000–47. doi:10.1210/er.2018-00275.
- Croce L, Gangemi D, Ancona G, et al. The cytokine storm and thyroid hormone changes in COVID-19. J Endocrinol Invest. 2021;44(5):891–904. doi:10.1007/s40618-021-01506-7.
- Pantos C, Tseti I, Mourouzis I. Use of triiodothyronine to treat critically ill COVID-19 patients: a new clinical trial. Crit Care. 2020;24(209):1–2. doi:10.1186/s13054-020-02934-2.
- Padhi R, Kabi S, Panda BN, Jagati S. Prognostic significance of nonthyroidal illness syndrome in critically ill adult patients with sepsis. Int J Crit Illn Inj Sci. 2018;8(3):165–72. doi:10.4103/IJCIIS.IJCIIS_29_17.
- Guo J, Hong Y, Wang Z, Li Y. Prognostic value of thyroid hormone FT3 in general patients admitted to the intensive care unit. Biomed Res Int. 2020;2020. doi:10.1155/2020/6329548.
- Ataoğlu HE, Ahbab S, Serez MK, et al. Prognostic significance of high free T4 and low free T3 levels in non-thyroidal illness syndrome. Eur J Intern Med. 2018;57:91–5. doi:10.1016/j.ejim.2018.07.018.
- Güven M, Gültekin H. The prognostic impact of thyroid disorders on the clinical severity of COVID-19: Results of single-centre pandemic hospital. Int J Clin Pract. 2021;75(6):e14129. doi:10.1111/ijcp.14129. Journal of Restorative Medicine 2022; 12: page 19 Thyroid Hormones & Eating Disorders
- Jacobs A, Derese I, Vander Perre S, et al. Non-thyroidal illness syndrome in critically ill children: prognostic value and impact of nutritional management. Thyroid. 2019;29(4):480–92. doi:10.1089/thy.2018.0420.
- Peeters RP, Wouters PJ, van Toor H, et al. Serum 3,3′,5′-triiodothyronine (rT3) and 3,5,3′-triiodothyronine/rT3 are prognostic markers in critically ill patients and are associated with postmortem tissue deiodinase activities. J Clin Endocrinol Metab. 2005;90(8):4559–65. doi:10.1210/jc.2005-0535.
- Schulte C, Reinhardt W, Beelen D, et al. Low T3-syndrome and nutritional status as prognostic factors in patients undergoing bone marrow transplantation. Bone Marrow Transplantation. 1998;22(12):1171.
- Liu J, Wu X, Lu F, et al. Low T3 syndrome is a strong predictor of poor outcomes in patients with communityacquired pneumonia. Sci Rep. 2016;6:8. doi:10.1038/srep22271.
- Wang Y, Zhou S, Bao J, et al. Low T3 levels as a predictor marker predict the prognosis of patients with acute ischemic stroke. Int J Neurosci. 2017;127(7):559–66. doi:10.1080/00207454.2016.1211649.
- Yaylali O, Kirac S, Yilmaz M, et al. Does hypothyroidism affect gastrointestinal motility? Gastroenterol Res Pract. 2009;2009:7. doi:10.1155/2009/529802.
- Ebert EC. The thyroid and the gut. J Clin Gastroenterol. 2010;44(6):402–6. doi:10.1097/MCG.0b013e3181d6bc3e.
- Ishii S, Kamegai J, Tamura H, et al. Triiodothyronine (T3) stimulates food intake via enhanced hypothalamic AMP-activated kinase activity. Regul Pept. 2008;151(1– 3):164–9. doi:10.1016/j.regpep.2008.07.007.
- Kong WM, Martin NM, Smith KL, et al. Triiodothyronine stimulates food intake via the hypothalamic ventromedial nucleus independent of changes in energy expenditure. Endocrinology. 2004;145(11):5252–8. doi:10.1210/en.2004-0545.
- Somogyi V, Gyorffy A, Scalise TJ, et al. Endocrine factors in the hypothalamic regulation of food intake in females: a review of the physiological roles and interactions of ghrelin, leptin, thyroid hormones, oestrogen and insulin. Nutr Res Rev. 2011;24(01):132–54. doi:10.1017/S0954422411000035.
- Amin A, Dhillo WS, Murphy KG. The central effects of thyroid hormones on appetite. J Thyroid Res. 2011;2011:306510. doi:10.4061/2011/306510.
- Wronski ML, Tam FI, Seidel M, et al. Associations between pituitary-thyroid hormones and depressive symptoms in individuals with anorexia nervosa before and after weight-recovery. Psychoneuroendocrinology. 2022;137:105630. doi:10.1016/j.psyneuen.2021.105630.
- Fazeli PK, Klibanski A. Effects of anorexia nervosa on bone metabolism. Endocr Rev. 2018;39(6):895–910. doi:10.1210/er.2018-00063.
- Aschettino-Manevitz D, Ornstein RM, Sterling WM, et al. Triiodothyronine (T3) and metabolic rate in adolescents with eating disorders: Is there a correlation? Eat Weight Disord. 2012;17(4):e252–8. doi:10.3275/8756.
- Croxson MS, Ibbertson HK. Low serum triiodothyronine (T3) and hypothyroidism in anorexia nervosa. J Clin Endocrinol Metab. 1977;44(1):167–74. doi:10.1210/jcem-44-1-167.
- Støving RK. Anorexia nervosa and endocrinology: a clinical update. Eur J Endocrinol. 2019;180(1):R9–27. doi:10.1530/EJE-18-0596.
- Azizi F. Effect of dietary composition on fastinginduced changes in serum thyroid hormones and thyrotropin. Metabolism. 1978;27(8):935–42. doi:10.1016/0026-0495(78)90137-3.
- Levinson CA, Hunt RA, Keshishian AC, et al. Using individual networks to identify treatment targets for eating disorder treatment: a proof-of-concept study and initial data. J Eat Disord. 2021;9(1):1–18. doi:10.1186/s40337-021-00504-7.
- Langouche L, Jacobs A, Van den Berghe G. Nonthyroidal illness syndrome across the ages. J Endocr Soc. 2019;3(12):2313–25. doi:10.1210/js.2019-00325.
- Abdalla SM, Bianco AC. Defending plasma T3 is a biological priority. Clin Endocrinol (Oxf). 2014;81(5):633–41. doi:10.1111/cen.12538.
- Hoermann R, Midgley JEM, Larisch R, Dietrich JW. Relational stability in the expression of normality, variation, and control of thyroid function. Front Endocrinol. 2016;7:142. doi:10.3389/fendo.2016.00142.
- Bianco AC, Salvatore D, Gereben B, et al. Biochemistry, cellular and molecular biology, and physiological roles of the iodothyronine selenodeiodinases. Endocr Rev. 2002;23(1):38–89. doi:10.1210/edrv.23.1.0455.
- Holtorf K. Thyroid hormone transport into cellular tissue. J Restor Med. 2014;3(1):53–68. doi:10.14200/jrm.2014.3.0104.
- Holtorf K. Peripheral thyroid hormone conversion and its impact on TSH and metabolic activity. J Restor Med. 2014;3(1):30–52. doi:10.14200/jrm.2014.3.0103.
- Fliers E, Bianco AC, Langouche L, Boelen A. Thyroid function in critically ill patients. Lancet Diabetes Endocrinol. 2015;3(10):816–25.
- Peeters RP, Wouters PJ, Kaptein E, et al. Reduced activation and increased inactivation of thyroid hormone in tissues of critically ill patients. J Clin Endocrinol Metab. 2003;88(7):3202–11. doi:10.1210/jc.2002-022013.
- Midgley JEM, Toft AD, Larisch R, et al. Time for a reassessment of the treatment of hypothyroidism. BMC Endocr Disord. 2019;19(1):37. doi:10.1186/s12902-019-0365-4.
- Hoermann R, Midgley JEM, Larisch R, Dietrich JW. Individualised requirements for optimum treatment of hypothyroidism: complex needs, limited options. Drugs Context. 2019;8:212597. doi:10.7573/dic.212597.
- Idrees T, Palmer S, Maciel RMB, Bianco AC. Liothyronine and desiccated thyroid extract in the treatment of hypothyroidism. Thyroid. 2020;30(10):1399–413. doi:10.1089/thy.2020.0153.
- Toft A. Thyroid hormone replacement – a counterblast to guidelines. J R Coll Physicians Edinb. 2017;47(4):307–9. doi:10.4997/JRCPE.2017.401.
- Jonklaas J, Bianco AC, Cappola AR, et al. Evidencebased use of levothyroxine/liothyronine combinations in treating hypothyroidism: a consensus document. Eur Thyroid J. 2021;10(1):10–38. doi:10.1159/000512970.
- Pantos C, Mourouzis I. Translating thyroid hormone effects into clinical practice: the relevance of thyroid hormone receptor α1 in cardiac repair. Heart Fail Rev. 2015;20(3):273–82. doi:10.1007/s10741-014-9465-4.
- Cappola AR, Desai AS, Medici M, et al. Thyroid and cardiovascular disease: research agenda for enhancing knowledge, prevention, and treatment. Thyroid. 2019;29(6):760–77. doi:10.1089/thy.2018.0416.
- Cappola A. Developing Oral LT3 Therapy for Heart Failure with Preserved Ejection Fraction (HFpEF). NIH; 2020. Accessed February 18, 2021. https://clinicaltrials. gov/ct2/show/study/NCT04111536.
- Cappola A. Developing Oral LT3 Therapy for Heart Failure with Reduced Ejection Fraction (HFrEF). NIH; Accessed February 18, 2021. https://clinicaltrials. gov/ct2/show/study/NCT04112316.
- Pantos C, Apostolaki V, Kokkinos L, et al. Acute triiodothyronine treatment and red blood cell sedimentation rate (ESR) in critically ill COVID-19 patients: a novel association? Clin Hemorheol Microcirc. 2021;79(3):485–8. doi:10.3233/CH-211215.
- Panicker V, Saravanan P, Vaidya B, et al. Common variation in the DIO2 gene predicts baseline psychological well-being and response to combination thyroxine plus triiodothyronine therapy in hypothyroid patients. J Clin Endocrinol Metab. 2009;94(5):1623–9. doi:10.1210/jc.2008-1301.
- Mughal BB, Fini JB, Demeneix BA. Thyroiddisrupting chemicals and brain development: an update. Endocr Connect. 2018;7(4):R160–86. doi:10.1530/EC-18-0029.
- Romano RM, Oliveira JM de, Oliveira VM de, et al. Could glyphosate and glyphosate-based herbicides be associated with increased thyroid diseases worldwide? Front Endocrinol. 2021;12:627167. doi:10.3389/fendo.2021.627167.
- Russell W, Harrison RF, Smith N, et al. Free Triiodothyronine has a distinct circadian rhythm that is delayed but parallels thyrotropin levels. J Clin Endocrinol Metabol. 2008;93(6):2300–6. doi:10.1210/jc.2007-2674.
- Smith TS. Thyroid T3 secretion compensates for T4-T3 conversion. Thyroid Patients Canada. Published May 4, Accessed May 25, 2020. https://thyroidpatients. ca/2020/05/04/t3-compensates-conversion/.
- Pilo A, Iervasi G, Vitek F, et al. Thyroidal and peripheral production of 3,5,3′-triiodothyronine in humans by multicompartmental analysis. Am J Physiol Endocrinol Metab. 1990;258(4):E715–26. doi:10.1152/ajpendo.1990.258.4.E715.
- Hoermann R, Pekker M, Midgley JE, et al. Triiodothyronine secretion in early thyroid failure: the adaptive response of central feedforward control. Eur J Clin Invest. 2019;50:e13192. doi: https://doi.org/10.1111/eci.13192.
- Citterio CE, Veluswamy B, Morgan SJ, et al. De novo triiodothyronine formation from thyrocytes activated by thyroid-stimulating hormone. J Biol Chem. 2017;292(37):15434-15444. doi:10.1074/jbc. M117.784447.
- Gereben B, McAninch EA, Ribeiro MO, Bianco AC. Scope and limitations of iodothyronine deiodinases in hypothyroidism. Nat Rev Endocrinol. 2015;11(11):642–52. doi:10.1038/nrendo.2015.155.
- Bianco AC, Kim BW. Deiodinases: implications of the local control of thyroid hormone action. J Clin Invest. 2006;116(10):2571–9. doi:10.1172/JCI29812.
- Hoermann R, Midgley J, Larisch R, Dietrich JW. Integration of peripheral and glandular regulation of triiodothyronine production by thyrotropin in untreated and thyroxine-treated subjects. Horm Metab Res. 2015;47:674–80.
- Hoermann R, Midgley JEM, Larisch R, Dietrich JW. Homeostatic control of the thyroid–pituitary axis: perspectives for diagnosis and treatment. Front Endocrinol. 2015;6:177. doi:10.3389/fendo.2015.00177.
- Peterson SJ, McAninch EA, Bianco AC. Is a normal TSH synonymous with “euthyroidism” in levothyroxine monotherapy? J Clin Endocrinol Metab. 2016;101(12):4964–73. doi:10.1210/jc.2016-2660.
- Gullo D, Latina A, Frasca F, et al. Levothyroxine monotherapy cannot guarantee euthyroidism in all athyreotic patients. PLoS One. 2011;6(8):e22552. doi:10.1371/journal.pone.0022552.
- Escobar-Morreale HF, Obregon MJ, Del Rey FE, De Escobar GM. Replacement therapy for hypothyroidism with thyroxine alone does not ensure euthyroidism in all tissues, as studied in thyroidectomized rats. J Clin Invest. 1995;96(6):2828–38.
- Jonklaas J, Bianco AC, Bauer AJ, et al. Guidelines for the treatment of hypothyroidism: prepared by the american thyroid association task force on thyroid hormone replacement. Thyroid. 2014;24(12):1670–751. doi:10.1089/thy.2014.0028.
- Piantanida E, Ippolito S, Gallo D, et al. The interplay between thyroid and liver: implications for clinical practice. J Endocrinol Invest. 2020;43(7):885–99. doi:10.1007/s40618-020-01208-6.
- Brechmann T, Sperlbaum A, Schmiegel W. Levothyroxine therapy and impaired clearance are the strongest contributors to small intestinal bacterial Journal of Restorative Medicine 2022; 12: page 21 Thyroid Hormones & Eating Disorders overgrowth: results of a retrospective cohort study. World J Gastroenterol. 2017;23(5):842–52. doi:10.3748/wjg. v23.i5.842.
- Gottwald-Hostalek U, Uhl W, Wolna P, Kahaly GJ. New levothyroxine formulation meeting 95–105% specification over the whole shelf-life: results from two pharmacokinetic trials. Curr Med Res Opin. 2017;33(2):169–74. doi:10.1080/03007995.2016.1246434.
- Huang SA, Bianco AC. Reawakened interest in type III iodothyronine deiodinase in critical illness and injury. Nat Clin Pract Endocrinol Metab. 2008;4(3):148–55. doi:10.1038/ncpendmet0727.
- Peeters RP, Visser TJ. Metabolism of Thyroid Hormone. MDText.com, Inc.; 2017. Accessed November 21, 2019. https://www.ncbi.nlm.nih.gov/books/NBK285545/.
- Howard D, Rosa FGL, Huang S, et al. Clinical case seminar: consumptive hypothyroidism resulting from hepatic vascular tumors in an athyreotic adult. J Clin Endocrinol Metab. 2011;96(7):1966. doi:10.1210/jc.2010-2104.
- Fliers E, Boelen A. An update on non-thyroidal illness syndrome. J Endocrinol Invest. 2021;44:1597–607. doi:10.1007/s40618-020-01482-4.
- Ohmori M, Harada K, Tsuruoka S, et al. Levothyroxineinduced liver dysfunction in a primary hypothyroid patient. Endocr J. 1999;46(4):579–83. doi:10.1507/endocrj.46.579.
- Kawakami T, Tanaka A, Negoro S, et al. Liver injury induced by levothyroxine in a patient with primary hypothyroidism. Intern Med. 2007;46(14):1105–8. doi:10.2169/internalmedicine.46.0086.
- Yu H, Zhang W, Shen C, et al. Liver dysfunction induced by Levothyroxine Sodium Tablets (Euthyrox®) in a hypothyroid patient with Hashimoto’s thyroiditis: case report and literature review. Endocr J. 2019;66(9):769–75. doi:10.1507/endocrj.EJ19-0078.
- Levothyroxine - FDA prescribing information, side effects and uses. Drugs.com. Accessed November 18, https://www.drugs.com/pro/levothyroxine.html.
- Peterson RE. The influence of the thyroid on adrenal cortical function. J Clin Invest. 1958;37(5):736–43. doi:10.1172/JCI103659.
- Hellman L, Bradlow HL, Zumoff B, Gallagher TF. The influence of thyroid hormone on hydrocortisone production and metabolism. J Clin Endocrinol Metab. 1961;21(10):1231–47. doi:10.1210/jcem-21-10-1231.
- Gordon GG, Southren AL. Thyroid-hormone effects on steroid-hormone metabolism. Bull NY Acad Med. 1977;53(3):241–59. Accessed April 25, 2021. https://www.ncbi.nlm.nih.gov/pmc/articles/PMC1807335/.
- Jones C. Metabolized Versus Free Cortisol: Understanding the Difference. Published online September 25, 2017. https://dutchtest.com/2017/09/25/metabolized-versus-free-cortisol-understanding-thedifference/.
- Hoshiro M, Ohno Y, Masaki H, et al. Comprehensive study of urinary cortisol metabolites in hyperthyroid and hypothyroid patients. Clin Endocrinol. 2006;64(1):37–45. doi:10.1111/j.1365-2265.2005.02412.x.
- Taniyama M, Honma K, Ban Y. Urinary cortisol metabolites in the assessment of peripheral thyroid hormone action: application for diagnosis of resistance to thyroid hormone. Thyroid. 1993;3(3):229–33. doi:10.1089/thy.1993.3.229.
- Nayer PD, Dozin B, Vandeput Y, et al. Altered interaction between triiodothyronine and its nuclear receptors in absence of Cortisol: a proposed mechanism for increased thyrotropin secretion in corticosteroid deficiency states. Eur J Clin Invest. 1987;17(2):106–10. doi:10.1111/j.1365-2362.1987.tb02388.x.
- Cremaschi GA, Gorelik G, Klecha AJ, et al. Chronic stress influences the immune system through the thyroid axis. Life Sci. 2000;67(26):3171–9. doi:10.1016/S0024-3205(00)00909-7.
- Usdan LS, Khaodhiar L, Apovian CM. The endocrinopathies of anorexia nervosa. Endocr Pract. 2008;14(8):1055–63. Accessed November 29, https://www.ncbi.nlm.nih.gov/pmc/articles/PMC3278909/.
- Misra M, Miller KK, Almazan C, et al. Alterations in cortisol secretory dynamics in adolescent girls with anorexia nervosa and effects on bone metabolism. J Clin Endocrinol Metab. 2004;89(10):4972–80. doi:10.1210/jc.2004-0723.
- Misra M, Klibanski A. Neuroendocrine consequences of anorexia nervosa in adolescents. Endocr Dev. 2010;17:197–214. doi:10.1159/000262540.
- Barnes BO, Galton L. Hypothyroidism: The Unsuspected Illness. Harper & Row New York; 1976.
- Hotze SF. Hypothyroidism, Health, and Happiness: The Riddle of Illness Revealed. Advantage Media Group; 2013.
- Starr M, Tennant JL. Hypothyroidism Type 2: The Epidemic. Mark Starr Trust; 2005.
- Robinson P. Recovering with T3: My Journey from Hypothyroidism to Good Health Using the T3 Thyroid Hormone. Elephant in the Room Books; 2018. Accessed March 1, 2020. http://recoveringwitht3.com/book/recovering-t3-my-journey-hypothyroidism-good-healthusing- t3-thyroid-hormone-0.
- Robinson P. Circadian T3 Method and Weaning Adrenal Steroids. Paul Robinson Thyroid. Published July 2, 2012. https://paulrobinsonthyroid.com/circadian-t3-method-and-weaning-adrenal-steroids/.
- Levine DT. The definition of Optimal Metabolism and its association with large reductions in chronic diseases. Trends Med. 2018;18(1). doi:10.15761/TiM.1000122.
- Baisier WV, Hertoghe J, Eeckhaut W. Thyroid insufficiency. Is thyroxine the only valuable drug? J Nutr Environ Med. 2001;11(3):159–66. doi:10.1080/13590840120083376. Journal of Restorative Medicine 2022; 12: page 22 Thyroid Hormones & Eating Disorders
- Bowthorpe J. Dosing with T3-only (whether by itself, or in combination with other thyroid meds). Stop The Thyroid Madness. Accessed March 31, 2021. https://stopthethyroidmadness.com/dosing-with-t3-only/.
- Bowthorpe J. Stop the Thyroid Madness: A Patient Revolution Against Decades of Inferior Treatment. Updated Revised. Laughing Grape Publishing; 2019.
- Morton JH. Sodium liothyronine in metabolic insufficiency syndrome and associated disorders: preliminary report. JAMA. 1957;165(2):124–9. doi:10.1001/jama.1957.02980200004002.
- Wilson DE. Reversing hypometabolic symptoms by normalizing low body temperatures with sustainedrelease T3 in patients with euthyroid TSH levels. J Restor Med. 2012;1(1):64–74. doi:10.14200/jrm.2012.1.1006.
- Friedman M, Miranda-Massari JR, Gonzalez MJ. Supraphysiological cyclic dosing of sustained release T3 in order to reset low basal body temperature. Puerto Rico Health Sci J. 2006;25(1):23–9.
- Thyroid Patients Canada. Thyroid patients are routinely excluded from low T3 syndrome (NTIS) research. Thyroid Patients Canada. Published August 28, 2019. Accessed November 7, 2019. https://thyroidpatients. ca/2019/08/27/thyroid-patients-are-routinely-excludedfrom- low-t3-syndrome-research/.
- Stanculescu D, Larsson L, Bergquist J. Theory: treatments for prolonged ICU patients may provide new therapeutic avenues for myalgic encephalomyelitis/chronic fatigue syndrome (ME/CFS). Front Med (Lausanne). 2021;8:672370. doi:10.3389/fmed.2021.672370.
- Adler SM, Wartofsky L. The nonthyroidal illness syndrome. Endocrinol Metab Clin North Am. 2007;36(3):657–72. doi:10.1016/j.ecl.2007.04.007.
- Santi A, da Cruz IBM, Loro VL, et al. Overt hypothyroidism is associated with blood inflammatory biomarkers dependent of lipid profile. J Appl Biomed. 2016;14(2):119–24. doi:10.1016/j.jab.2015.10.002.
- Mancini A, Di Segni C, Raimondo S, et al. Thyroid hormones, oxidative stress, and inflammation. Mediators Inflamm. 2016;2016:12. doi:10.1155/2016/6757154.
- Stanculescu D, Larsson L, Bergquist J. Hypothesis: mechanisms that prevent recovery in prolonged ICU patients also underlie myalgic encephalomyelitis/chronic fatigue syndrome (ME/CFS). Front Med. 2021;8:628029. doi:10.3389/fmed.2021.628029.
- Scanelli G, Schlagenauf P, Degli Esposti L. Endocrine Abnormalities in Eating Disorders. In: Eating Disorders and the Skin. Springer, Berlin, Heidelberg; 2013. pp. 31–6.
- Troisi A, Di Lorenzo G, Lega I, et al. Plasma ghrelin in anorexia, bulimia, and binge-eating disorder: relations with eating patterns and circulating concentrations of cortisol and thyroid hormones. Neuroendocrinology. 2005;81:259–66.
- Fröhlich E, Wahl R. Microbiota and thyroid interaction in health and disease. Trends Endocrinol Metab. 2019;30(8):479–90. doi:10.1016/j.tem.2019.05.008.
- Knezevic J, Starchl C, Tmava Berisha A, Amrein K. Thyroid-gut-axis: how does the microbiota influence thyroid function? Nutrients. 2020;12(6):1769. doi:10.3390/nu12061769.
- Litwińczuk M, Szydełko J, Szydełko M. The role of gut microbiota in patients with autoimmune thyroid diseases–current status and future perspectives. J Educ Health Sport. 2019;9(9):816–27. doi:10.5281/zenodo.3460391.
- Aceves C, Mendieta I, Anguiano B, Delgado-González E. Molecular iodine has extrathyroidal effects as an antioxidant, differentiator, and immunomodulator. Int J Mol Sci. 2021;22(3):1228. doi:10.3390/ijms22031228.
- De la Vieja A, Santisteban P. Role of iodide metabolism in physiology and cancer. Endocr Relat Cancer. 2018;25(4):R225–45. doi:10.1530/ERC-17-0515.
- Abraham GE, Flechas JD, Hakala JC. Orthoiodosupplementation: Iodine sufficiency of the whole human body. Orig Intern. 2002;9(4):30–41. https://www.optimox.com/iodine-study-2.
- Brownstein D. Clinical experience with inorganic, nonradioactive iodine/iodide. Orig Intern. 2005;12(3):105–8. https://www.optimox.com/iodine-study-9.
- Gärtner R, Gasnier BCH, Dietrich JW, et al. Selenium supplementation in patients with autoimmune thyroiditis decreases thyroid peroxidase antibodies concentrations. J Clin Endocrinol Metab. 2002;87(4):1687–91. doi:10.1210/jcem.87.4.8421.
- Brownstein D. Iodine: Why You Need It, Why You Can’t Live Without It. 5th ed. Medical Alternative Press; 2014.
- Hotz CS, Fitzpatrick DW, Trick KD, L’Abbé MR. Dietary iodine and selenium interact to affect thyroid hormone metabolism of rats. J Nutr. 1997;127(6):1214–8. doi:10.1093/jn/127.6.1214.
- Dahiya K, Verma M, Dhankhar R, et al. Thyroid profile and iron metabolism: mutual relationship in hypothyroidism. Biomed Res. 2016;27(4).
- Eftekhari MH, Keshavarz SA, Jalali M, et al. The relationship between iron status and thyroid hormone concentration in iron-deficient adolescent Iranian girls. Asia Pac J Clin Nutr. 2006;15(1):50–5.
- Bowthorpe J. Hashimoto’s: Taming the Beast. Laughing Grape Publishing; 2019.
- Roberts PL. Thyroid toxicity. In: Bowthorpe J, ed. Stop the Thyroid Madness II: How Thyroid Experts Are Challenging Ineffective Treatments and Improving the Lives of Patients. Florence: Grape Publishing; 2011. pp. 215–44.
- Evliyaoğlu O, Acar M, Özcabı B, et al. Vitamin D deficiency and Hashimoto’s thyroiditis in children and adolescents: a critical vitamin D level for Journal of Restorative Medicine 2022; 12: page 23 Thyroid Hormones & Eating Disorders this association? J Clin Res Pediatr Endocrinol. 2015;7(2):128–33. doi:10.4274/jcrpe.2011.
- Kunc M, Gabrych A, Witkowski JM. Microbiome impact on metabolism and function of sex, thyroid, growth and parathyroid hormones. Acta Biochim Pol. 2016;63(2):189–201. doi:10.18388/abp.2015_1093.
- Smitka K, Prochazkova P, Roubalova R, et al. Current aspects of the role of autoantibodies directed against appetite-regulating hormones and the gut microbiome in eating disorders. Front Endocrinol. 2021;12:293. doi:10.3389/fendo.2021.613983.
- Parker A, Fonseca S, Carding SR. Gut microbes and metabolites as modulators of blood-brain barrier integrity and brain health. Gut Microbes. 2020;11(2):135–57. doi:10.1080/19490976.2019.1638722.
- Daher R, Yazbeck T, Jaoude JB, Abboud B. Consequences of dysthyroidism on the digestive tract and viscera. World J Gastroenterol. 2009;15(23):2834–8. doi:10.3748/wjg.15.2834.
- Yago MAR, Frymoyer AR, Smelick GS, et al. Gastric Re-acidification with betaine HCl in healthy volunteers with rabeprazole-induced hypochlorhydria. Mol Pharm. 2013;10(11):4032–7. doi:10.1021/mp4003738.
- Kines K, Krupczak T. Nutritional interventions for gastroesophageal reflux, irritable bowel syndrome, and hypochlorhydria: a case report. Integr Med (Encinitas). 2016;15(4):49–53. Accessed April 25, https://www.ncbi.nlm.nih.gov/pmc/articles/PMC4991651/.
- Han H, Yi B, Zhong R, et al. From gut microbiota to host appetite: gut microbiota-derived metabolites as key regulators. Microbiome. 2021;9(1):162. doi:10.1186/s40168-021-01093-y.
- Zanchi D, Depoorter A, Egloff L, et al. The impact of gut hormones on the neural circuit of appetite and satiety: a systematic review. Neurosci Biobehav Rev. 2017;80:457–75.
- Begg DP, Woods SC. The endocrinology of food intake. Nat Rev Endocrinol. 2013;9(10):584–97. doi:10.1038/nrendo.2013.136.
- Fetissov SO. Role of the gut microbiota in host appetite control: bacterial growth to animal feeding behaviour. Nat Rev Endocrinol. 2017;13(1):11–25. doi:10.1038/nrendo.2016.150.
- Santini F, Marzullo P, Rotondi M, et al. The crosstalk between thyroid gland and adipose tissue: signal integration in health and disease. Eur J Endocrinol. 2014;171(4):R137–52. doi:10.1530/EJE-14-0067.
- Rosenbaum M, Goldsmith RL, Haddad F, et al. Triiodothyronine and leptin repletion in humans similarly reverse weight-loss-induced changes in skeletal muscle. Am J Physiol Endocrinol Metab. 2018;315(5):E771–9. doi:10.1152/ajpendo.00116.2018.
- Kahraman H, Kaya N, Demirçali A, et al. Gastric emptying time in patients with primary hypothyroidism. Eur J Gastroenterol Hepatol. 1997;9(9):901–4. doi:10.1097/00042737-199709000-00014.
- Araujo RL, Andrade BM, da Silva ML, et al. Tissuespecific deiodinase regulation during food restriction and low replacement dose of leptin in rats. Am J Physiol Endocrinol Metab. 2009;296(5):E1157–63.
- Leibel RL, Hirsch J. Diminished energy requirements in reduced-obese patients. Metab Clin Exp. 1984;33(2):164–70. doi:10.1016/0026-0495(84)90130-6.
- Ferrell JE. Self-perpetuating states in signal transduction: positive feedback, double-negative feedback and bistability. Curr Opin Cell Biol. 2002;14(2):140–8. doi:10.1016/S0955-0674(02)00314-9.
- Angeli D, Ferrell JE, Sontag ED. Detection of multistability, bifurcations, and hysteresis in a large class of biological positive-feedback systems. Proc Natl Acad Sci USA. 2004;101(7):1822–7. doi:10.1073/pnas.0308265100.
- Berberich J, Dietrich JW, Hoermann R, Müller MA. Mathematical modeling of the pituitary–thyroid feedback loop: role of a TSH-T3-shunt and sensitivity analysis. Front Endocrinol. 2018;9. doi:10.3389/fendo.2018.00091.
- Hall RCW, Popkin MK, Devaul R, et al. Psychiatric manifestations of Hashimoto’s thyroiditis. Psychosomatics. 1982;23(4):337–42. doi:10.1016/S0033-3182(82)73397-3.
- Siegmann EM, Müller HHO, Luecke C, et al. Association of depression and anxiety disorders with autoimmune thyroiditis: a systematic review and metaanalysis. JAMA Psychi. 2018;75(6):577–84. doi:10.1001/jamapsychiatry.2018.0190.
- Pehlivantürk Kızılkan M, Kanbur N, Akgül S, Alikaşifoğlu A. An adolescent boy with comorbid anorexia nervosa and hashimoto thyroiditis. J Clin Res Pediatr Endocrinol. 2016;8(1):92–5. doi:10.4274/jcrpe.2297.
- Lakhani OJ, Tripathi S, Indu KC, Desai M. Levothyroxine replacement before glucocorticoid replacement leading to adrenal crisis in a case of autoimmune polyendocrine syndrome type II (Schmidt syndrome). Thyroid Res Pract. 2015;12(3):116. doi:10.4103/0973-0354.157932.
- Hoermann R, Midgley J, Larisch R, Dietrich JW. Is pituitary thyrotropin an adequate measure of thyroid hormone-controlled homeostasis during thyroxine treatment? Eur J Endocrinol. 2013;168(2):271–80. doi:10.1530/EJE-12-0819.
- Fraser WD, Biggart EM, O’Reilly DS, et al. Are biochemical tests of thyroid function of any value in monitoring patients receiving thyroxine replacement? Br Med J (Clin Res Ed). 1986;293(6550):808–10.
- Chedid V, Dhalla S, Clarke JO, et al. Herbal therapy is equivalent to rifaximin for the treatment of small intestinal bacterial overgrowth. Glob Adv Health Med. 2014;3(3):16–24. doi:10.7453/gahmj.2014.019. Journal of Restorative Medicine 2022; 12: page 24 Thyroid Hormones & Eating Disorders
- Sachdev AH, Pimentel M. Gastrointestinal bacterial overgrowth: pathogenesis and clinical significance. Ther Adv Chronic Dis. 2013;4(5):223–31. doi:10.1177/2040622313496126.
- Severo JS, Morais JBS, de Freitas TEC, et al. The role of zinc in thyroid hormones metabolism. Int J Vitam Nutr Res. 2019;89(1–2):80–8. doi:10.1024/0300-9831/a000262.
- Sworczak K, Wiśniewski P. The role of vitamins in the prevention and treatment of thyroid disorders. Endokrynol Pol. 2011;62(4):340–4.
- The Importance of Iron and Ferritin in Hypothyroidism. Thyroid UK. Published September 24, 2019. Accessed November 13, https://thyroiduk.org/if-you-are-hypothyroid/importance-of-iron-and-ferritin-in-hypothyroidism/.